Research Overview before 2005
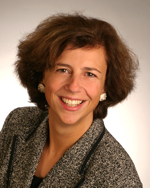
Prof. Dr. Ursula Keller, ETH Zurich, Switzerland
also available as DownloadMS-Word (DOC, 301 KB)vertical_align_bottom and as Downloadpdf file (PDF, 74 KB)vertical_align_bottom.
Main research areas
Ultrafast lasers based on passively modelocked diode-pumped solid-state and
semiconductor lasers using semiconductor saturable absorber mirrors (SESAMs),
ultrashort pulse generation in the one to two optical cycle regime,
frequency combs and carrier-envelope offset (CEO) stabilization,
reliable and functional instrumentation for extreme ultraviolet (EUV) generation and
attosecond soft-X-ray experiments using high harmonic generation,
attosecond science
A) Ultrafast diode-pumped lasers:
We have made key contributions to frontiers in the performance of ultrafast lasers and their improvement through her contribution to the understanding of Kerr lens modelocking (KLM) [1] and the invention and development of semiconductor saturable absorbers mirrors (SESAM devices) [2-4], a novel family of optical devices that allow for very simple, self-starting passive modelocking of ultrafast solid-state and semiconductor lasers [5-6] and Q-switching of microchip lasers [7-9]. The multi-disciplinary work involving understanding of both semiconductor materials and devices plus solid-state laser physics [10-12] has resulted in new unprecedented performance improvements in terms of pulse widths, average power, and repetition rates in these lasers. Previously, Q-switching instabilities prevented stable passive modelocking of solid-state lasers for more than 25 years [13]. In addition, KLM has significant limitations, since it is generally not self-starting, and critical cavity alignment close to the stability limit is required. Thus, passively modelocked solid-state lasers using intracavity SESAMs have become a very attractive alternative to KLM, and are today widely used in the above 10-fs regime in both research and commercial environments [14]. SESAM modelocked solid-state lasers have increased pulse energies by three orders of magnitude, up to ≈5 µJ [15], and increased pulse repetition rates by more than two orders of magnitude, to 160 GHz [16-17].
Extending this technique to passively modelocked optically pumped semiconductor (OPS) lasers based on vertical external cavity surface emitting lasers (VECSELs) [18-20], we have pushed the performance of these types of lasers into the multi-watt level based on new concepts and devices – again more than two orders of magnitude improvement [21-23]. Wafer-scale integration will make them extremely attractive for high-volume applications [24].
- Download[17] (PDF, 949 KB)vertical_align_bottom Optics Lett., vol. 16, pp. 1022-1024, 1991
- Download[19] (PDF, 1 MB)vertical_align_bottom Optics Lett., vol. 17, pp. 505-507, 1992
- Download[62] (PDF, 738 KB)vertical_align_bottom IEEE J. Selected Topics in Quantum Electronics (JSTQE), vol. 2, pp. 435-453, 1996
- Download[228] (PDF, 157 KB)vertical_align_bottom Appl. Phys. B, vol. 81, Nr. 1, pp. 27-32, 2005
- Download[185] (PDF, 1.6 MB)vertical_align_bottom Nature, vol. 424, pp. 831-838, 2003
- Progress in Optics, vol. 46, pp. 1-115, 2004
- Download[93] (PDF, 671 KB)vertical_align_bottom J. Opt. Soc. Am. B, vol. 16, pp. 376-388, 1999
- Download[152] (PDF, 280 KB)vertical_align_bottom Appl. Phys. B, vol. 72, pp. 285-287, 2001
- Download[154] (PDF, 359 KB)vertical_align_bottom J. Opt. Soc. Am. B, vol. 18, pp. 1805-1812, 2001
- Download[39] (PDF, 336 KB)vertical_align_bottom Optics Lett., vol. 20, pp. 16-18, 1995
- IEEE J. Selected Topics in Quantum Electronics (JSTQE), vol. 2, pp. 540-556, 1996
- Download[156] (PDF, 137 KB)vertical_align_bottom Appl. Phys. B, vol. 73, pp. 653-662, 2001
- Download[90] (PDF, 829 KB)vertical_align_bottom J. Opt. Soc. Am. B, vol. 16, pp. 46-56, 1999
- see extensive tables in Progress in Optics, vol. 46, pp. 1-115, 2004
- Download[179] (PDF, 95 KB)vertical_align_bottom Optics Lett., vol. 28, pp. 367-369, 2003 and recently Postdeadline paper at ASSP 2006
- vol. 38, pp. 1331-1338, 2002
- Download[211] (PDF, 248 KB)vertical_align_bottom New Journal of Physics, vol. 6, paper 174 (13 pages), 2004
- Download[134] (PDF, 130 KB)vertical_align_bottom IEEE Photonics Tech. Lett., vol. 12, pp. 1135-1137, 2000
- Download[177] (PDF, 215 KB)vertical_align_bottom Appl. Phys. B, vol. 75, pp. 445-451, 2002
- Download[172] (PDF, 362 KB)vertical_align_bottom IEEE J. Quantum Electron., vol. 38, pp. 1268-1275, 2002
- Download[219] (PDF, 712 KB)vertical_align_bottom Optics Lett., vol. 30, pp. 272-274, 2005
- Download[222] (PDF, 157 KB)vertical_align_bottom Appl. Phys. Lett., vol. 86, 131102, 2005
- Physics Reports, invited review article submitted Dec. 8, 2005
- Download[207] (PDF, 518 KB)vertical_align_bottom Appl. Phys. B, vol. 79, Nr. 8, pp. 927-932, 2004
B) Ultrashort pulses with new world-record pulse durations in the regime of one to two optical cycles:
We have demonstrated ultrashort pulses of only about two optical cycles (i.e. ≈5 fs pulse duration) directly from KLM Ti:sapphire lasers [1,2] and with external pulse compression using supercontinuum generation in microstructure fibers [3]. We have pushed world-record performance using specially designed double chirped mirrors for dispersion compensation [4-6]. This opens up a completely new regime where the exact position of the electric field underneath the pulse envelope (i.e. the carrier envelope offset – CEO) in nonlinear optics has become important and relevant.
- Download[99] (PDF, 330 KB)vertical_align_bottom Optics Lett., vol. 24, pp. 631-633, 1999
- Science, vol. 286, pp. 1507-1512, 1999
- Download[221] (PDF, 427 KB)vertical_align_bottom J. Opt. Soc. Am. B, vol. 22, pp. 687-693, 2005
- Download[69] (PDF, 330 KB)vertical_align_bottom Optics Lett., vol. 22, pp. 831-833, 1997
- Download[92] (PDF, 824 KB)vertical_align_bottom IEEE J. Quantum Electronics, vol. 35, pp. 129-137, 1999
- Download[135] (PDF, 1.8 MB)vertical_align_bottom Appl. Phys. B, vol. 71, pp. 509-522, 2000
C) Carrier envelope offset (CEO) control and stabilization using frequency combs:
Inside a femtosecond laser oscillator, no stabilization mechanism between the propagation speeds of the carrier and the pulse envelope exists. Therefore, the relative delay between carrier and envelope of a femtosecond oscillator will exhibit irregular fluctuations unless this jitter is actively suppressed. We have introduced and made first feasibility demonstrations for several novel techniques to measure and stabilize the CEO fluctuations in the frequency domain using the frequency comb generated by the modelocked oscillator [1]. For example we proposed both the f-to-2f and the 2f-to-3f heterodyne technique [1] which are being used today. Our pioneering contribution to this field was also recognized by the Nobel committee for the Physics Nobel Prize in 2005 (see page 11, Ref. 37, "Advanced Information" for the Nobel Prize in Physics 2005, download from here. Both intensity and beam pointing fluctuations in the laser can introduce carrier-envelope phase changes. We have discussed the physical origin of the CEO fluctuations and have optimized the laser cavity to stabilize the carrier envelope-phase with a long-term residual jitter corresponding to only 10 attoseconds in a (100 kHz – 0.01 Hz) bandwidth [2]. Currently we are exploring different more compact sources (i.e. not based on Ti:sapphire lasers) that could generate frequency combs for optical communication and metrology applications.
- Download[113] (PDF, 405 KB)vertical_align_bottom Appl. Phys. B, vol. 69, pp. 327-332, 1999
- Download[188] (PDF, 1.2 MB)vertical_align_bottom IEEE J. of Sel. Top. in Quantum Electron., vol. 9, No. 4, pp. 1030-1040, 2003
D) Intense few-cycle pulse generation:
Single attosecond pulse generation requires not only intense, reproducible few-cycle drive pulses, but also a control of the carrier phase with respect to the pulse envelope. Until recently the only successful demonstration of CEO-phase-locked intense pulses with pulse energies of a few 100 µJ was based on a CEO stabilized Ti:sapphire laser, chirped-pulse amplification and pulse compression in a hollow fiber, with which we have demonstrated pulses as short as 3.8 fs [1]. Meanwhile, we have demonstrated two new promising ways to achieve this goal. One is based on chirped pulse optical parametric amplification (CPOPA) [2] and the other on pulse compression through filamentation [3]. We have demonstrated the generation of intense CEO-phase stabilized 5.1-fs pulses by filamentation in an excellent spatial mode [4]. The filament will be the most promising approach for pulse compression into the single cycle regime. In contrast to the hollow fiber approach, this is a self-compression scheme which ultimately should go beyond the bandwidth limitations of chirped mirrors [5,6].
- Download[187] (PDF, 471 KB)vertical_align_bottom Optics Lett., vol. 28, pp. 1987-1989, 2003
- Download[197] (PDF, 1.6 MB)vertical_align_bottom Optics Lett., vol. 29, pp. 1369-1371, 2004
- Download[206] (PDF, 239 KB)vertical_align_bottom Appl. Phys. B, vol. 79, Nr. pp. 673-677, 2004
- Download[234] (PDF, 534 KB)vertical_align_bottom Opt. Express, vol. 13, No. 19, pp. 7541-7547, 2005
- Download[236] (PDF, 169 KB)vertical_align_bottom Optics Lett., vol. 30, pp. 2657-2659, 2005
- Download[241] (PDF, 716 KB)vertical_align_bottom Journal of Modern Optics, vol. 53, pp. 75-85, 2006
E) High harmonic generation and attosecond science:
For longer intense infrared (IR) pulses focused into a gas target, attosecond pulse trains (APTs) can be generated instead of a single attosecond pulse. We have proposed [1] and are in the process of demonstrating the possibility for coherent control of high-order harmonic generation using APTs. Here the APTs are being used as a tool for controlling strong field processes in high-order harmonic generation. When used in combination with an intense IR laser field, the timing of the APT with respect to the IR laser field can be used to microscopically select a single quantum path contribution to a process that would otherwise consist of many interfering components. It is through this timing that we predict control over the release of the electron into the continuum, its excursion inside the continuum and consequently influence the yield and coherence of the harmonics. Macroscopic propagation effects in the APT-assisted harmonics generation predicts a much larger enhancement than by the simple single atom model alone [2]. This is a volume effect, because the enhancement is much larger at the lower IR intensity. In a first experiment, we have generated extreme ultraviolet (XUV) harmonics around 90 eV in helium using a combination of vacuum ultraviolet (VUV) harmonics, generated in a Xe capillary, and the strong IR laser pulse. With no changes in the IR input energy or the configuration of the helium target, the collinearly focused combination of the two fields changed the spectral properties and increased the yield of the XUV harmonics compared to those generated with the IR field alone [3].
- Download[190] (PDF, 243 KB)vertical_align_bottom Phys. Rev. Lett., vol. 92, No. 2, 023003, 16. Jan. 2004
- Download[231] (PDF, 536 KB)vertical_align_bottom Phys. Rev. A, vol. 72, 013411, 2005
- Download[242] (PDF, 1.4 MB)vertical_align_bottom Journal of Modern Optics, vol. 53, pp. 87-96, 2006
F) Ultrafast semiconductor spectroscopy and ultrafast SNOM:
We explored several important and interesting topics such as ultrafast dephasing of continuum transitions [1] and optimization of semiconductor nonlinearitites with an adaptive feedback control [2,3]. Currently we mainly use special high-accuracy pump-probe techniques to characterize SESAMs [4] and optimize their performance with regards to different device structure [5,6], materials [7-9], nonlinearities [10], quantization levels, growth parameters [11] and post-growth processing [12]. We have one dedicated lab to perform these measurements. We built-up a state-of-the-art ultrafast SNOM [13] and we achieved some interesting results [14-16]. However, the spatial resolution was signal-to-noise limited to about 200 nm for nonlinear pump-probe measurements in semiconductor nanostructures. We would have expected to see more interesting physics on a much smaller scale but could not overcome the fundamental limitation due to surface effects that would dominate the nonlinear dynamics. There may be more oportunities for molecules, polymers and biology, however confocal microscopy will also compete against this approach.
- Download[101] (PDF, 150 KB)vertical_align_bottom Phys. Rev. B, vol. 59, pp. 14860-14863, 1999
- Download[132] (PDF, 494 KB)vertical_align_bottom Appl. Phys. Lett., vol. 77, pp. 924-926, 2000
- Download[147] (PDF, 322 KB)vertical_align_bottom J. Opt. Soc. Am. B, vol. 18, pp. 872-881, 2001
- Download[200] (PDF, 417 KB)vertical_align_bottom Appl. Phys. B, vol. 79, pp. 331-339, 2004
- Download[62] (PDF, 738 KB)vertical_align_bottom IEEE J. Selected Topics in Quantum Electronics (JSTQE), vol. 2, pp. 435-453, 1996
- Download[228] (PDF, 467 KB)vertical_align_bottom Appl. Phys. B, vol. 81, Nr. 1, pp. 27-32, 2005
- Download[235] (PDF, 121 KB)vertical_align_bottom GaInNAs at 1.3 µm: Appl. Phys. Lett. 84, 4002, 2004 and Appl. Phys. Lett. 87, 132103, 2005
- Download[223] (PDF, 86 KB)vertical_align_bottom GaInNAs at 1.5 µm: Electron. Lett., vol. 41, Nr. 6, pp. 321-323, 2005
- Download[209] (PDF, 91 KB)vertical_align_bottom AlGaAsSb: Electron. Lett. 40, Nr. 22, pp. 1414-1415, 2004 and IEEE Phot. Tech. Lett., accepted
- Download[215] (PDF, 922 KB)vertical_align_bottom Appl. Phys. B, vol. 80, pp. 151-158, 2005
- Download[100] (PDF, 154 KB)vertical_align_bottom LT growth: Appl. Phys. Lett. vol. 74, 3134, 1999, and vol. 74, 1269, 1999, and vol. 69, 2566, 1996
- Download[110] (PDF, 264 KB)vertical_align_bottom Appl. Phys. Lett., vol. 75, pp. 1437-1439, 1999 and Appl. Phys. Lett., vol. 74, pp. 1993-1995, 1999
- Download[102] (PDF, 297 KB)vertical_align_bottom Review of Scientific Instruments, vol. 70, pp. 2758-2764, 1999
- Download[107] (PDF, 169 KB)vertical_align_bottom Phys. Rev. B, vol. 60, pp. 2101-2105, 1999
- Download[121] (PDF, 242 KB)vertical_align_bottom Appl. Phys. Lett., vol. 76, pp. 2695-2697, 2000
- Download[166] (PDF, 561 KB)vertical_align_bottom Phys. Rev. B, vol. 65, 045322 , 2002